Summary
Modern physics had its beginnings about a century ago with the birth of quantum mechanics. Over the past few decades, scientists have built on knowledge of electromagnetism to master the laws of the weak and strong interactions. They interactions all follow the gauge theory first proposed by Yang Chen-Ning and Mills. The weak interaction distinguishes left from right, exhibiting the non-conservation of parity first proposed by Yang together with Lee Tsung-Dao. This article is an excerpt from a public lecture, "100 Years of Physics: Celebration for CN Yang at One Hundred". It sketches the main developments in physics over the past century, provides a brief history of Professor Yang’s life and his ground breaking works, and offers a look ahead to physics in the 21st century.
*This is an extract of Chinese version. For the full version (in Chinese only), please refer to Chinese page. Translation by the Council of the Hong Kong Laureate Forum.
Contents
- Introduction
-
Developments in physics over the past century
2.1 From classical to quantum physics
2.2 The composition of the nucleus and particle interactions -
Biography of Professor Yang Chen-Ning
3.1 Birth and early life
3.2 Study abroad and early research work
3.3 Relationship with CUHK
3.4 Return to Tsinghua -
Concluding remarks
1. Introduction
Professor Yang Chen-Ning is one of the most important theoretical physicists of the contemporary era, and one of the first Chinese to receive the Nobel Prize. He has also been a friend of The Chinese University of Hong Kong (CUHK) for more than half a century. As he enters the hundredth year of a remarkable life, the Chinese Physical Society, Tsinghua University and CUHK have jointly organised the "Celebratory Conference for CN Yang at One Hundred", held in Beijing on 22 and 23 September and attended online by friends and fellow physicists in Hong Kong. Unlike the conference targeting physicists, the seminar today aims to provide young Hong Kong students with some insight into the life and work of Professor Yang, and to look ahead to the future of physics in the next hundred years. Of course, ‘looking ahead to the future’ is a trite phrase often bandied about in many discussions, but the phrase is especially apt for today’s event, given that much of the progress in physics in the next one hundred years (mainly concerning elementary particles, but also extending to other fields) will inevitably be built upon on the non-commutative gauge theory pioneered by Professor Yang, just as all classical physics is built upon Newton's laws. The pivotal position of gauge theories is not well appreciated by the general public, and it will be one purpose of the present talk to explain the unique contributions of Professor Yang.
2. Developments in physics over the past century
2.1 From classical to quantum physics
The past century has seen a host of new discoveries in the field of physics. Today, I will focus on the most significant advances and hope to use a few well-known and tantalising formulas to highlight the key developments of different periods.
Modern physics (and even modern science) began with Galileo (1564-1642). From Galileo to the end of the 19th century was the era of classical physics. The iconic achievements during this period were Newton’s laws, especially the Second Law

as well as electromagnetism and the related (classical) field concepts. Everything else was but consequential and secondary. Even today, equation (1) is still the point from which all investigations in classical physics start.
However, by the end of the 19th century, classical physics was in crisis. Why are atoms stable? Why does the heat capacity of solids decrease sharply at low temperatures? Why don’t heated cavities emit copious amounts of ultraviolet light? Classical mechanics was unable to explain these phenomena, revealing inherent deficiencies and even internal inconsistencies.
It took about two decades to solve these problems. When the dust settled, Heisenberg and Born came up with an elegant formulation. If is a coordinate and
a momentum, then

Even primary school students would know that

Similarly, secondary school students with just a little bit of algebra would tell you that the answer to (2) is zero, and only a fool would pose such a "dumb" question. However, Heisenberg and Born were definitely not fools – they stated that

In this equation, in SI units, where
is Planck’s constant. For macroscopic objects,
is large, and
on the right can be ignored. This means that
and
can be regarded as the same, and we can apply the principles of classical physics and elementary school mathematics.
However, for microscopic systems such as atoms, is not (much) larger than
, and the right-hand side cannot be ignored, meaning that equation (3) has to be used. Intriguingly, the equation also contains
!
The principles behind this formula gave birth to quantum mechanics, which then provided a complete (and quantitative) explanation of the properties and laws of atoms, and resolved the questions that had plagued classical physics. As well as explaining phenomena in the realm of physics, quantum mechanics also had a big impact on chemistry.
It may be an exaggeration to say that all of our knowledge of chemistry and life sciences can be derived from equation (3). However, you can turn this statement around: without equation (3), we wouldn't understand chemistry, and without chemistry, we would not understand life sciences. In other words, equation (3) is a necessary (though not necessarily sufficient) condition for understanding chemistry and life sciences.
This equation was developed almost a century ago, in 1925. In the view of Kuhn in his study of scientific revolutions [1], the science of the following two decades consisted of nothing more than filling in details and dealing with the consequences of this new discovery. Of course, some of these "details" were also extraordinary in their own right, such as extending quantum mechanics to systems with infinite degrees of freedom (such as the electromagnetic field) and dealing with the resulting divergences, and the existence of antiparticles.
2.2 The composition of the nucleus and particle interactions
Beginning in the 1940s, especially after the end of World War II, physicists shifted their attention from atoms and molecules to the composition of the nucleus and the interactions among its components. All interactions can be divided into four categories: (1) the strong interaction that holds the nucleus together; (2) electromagnetism; (3) the weak interaction that causes decay; and (4) gravitation. Gravity is unimportant at the subatomic level (though it dominates astronomical phenomena), and is not easy to integrate with quantum mechanics (this remains an unsolved problem to the present day), so we won’t further discuss it below.
Electromagnetism
Among the other three fundamental interactions, electromagnetism was the first to be understood. In a sense, it is not even necessary to add any new concepts here; it suffices to consider Maxwell’s electromagnetic theory together with the quantum mechanics of Heisenberg, Schrödinger, and Dirac.
Electromagnetic theory uses the series expansion in powers of , where
is the dimensionless fine-structure constant.
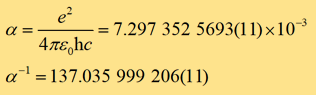
For example, the strength of the electron magnetic dipole moment can be expressed in terms of the dimensionless parameter , which can be written as

The problem is how to calculate the coefficients. As
, the series expansion is accurate (though not convergent). Dirac’s ingenious theory gives
. (For classical particles,
). However, when calculating
, one must sum over the infinite number of intermediate states in the process, giving the result
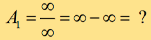
Schwinger, Tomonaga, and Feynman developed a renormalisation theory to enable these seemingly absurd formulas to yield unambiguous finite values, for example

The three scientists won the Nobel Prize in Physics two decades later. (With scientific interchange impossible during World War II, Schwinger and Tomonaga obtained their results independently using somewhat different methods. Feynman followed soon after; his approach showed the greatest clarity, and has since become the path adopted for use in higher-order computations.) Higher-order computation are very complex – with decades of work by dozens of scientists, results up towere obtained; when applied to the above equation, the series gives
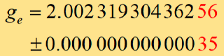
There are no adjustable parameters here. Theory and experiments agree to 13 significant figures – the most precise test in modern physics. Quantum electrodynamics can be regarded as fully resolved.
Recently, the same parameter for muons () has attracted attention due to discrepancies between the theoretical value and experimental results. However, this goes beyond quantum electrodynamics, as higher-order corrections involve the strong and weak interactions. (In fact, Dyson also pointed out a long time ago that the series expansion does not converge, and that there should be non-analytic corrections; however this has not been much discussed recently.)
Weak interactions
The weak interaction that causes decay is more elusive, but is now also well understood. The weak interaction has the following key properties:
- It acts only on left-handed particles. The force distinguishes between left and right, a possibility that was first proposed by Yang Chen-Ning and Lee Tsung-Dao in 1956 and confirmed during the course of the following year in an experiment by Chien-Shiung Wu and others.
- Left-handed particles are coupled with spin-1 bosons (this is the
theory proposed by Feynman and Gell-Mann; the main idea is also implicit in the theory proposed by Lee and Yang).
- However, the spin-1 boson theory diverges, and presents significantly greater challenges than quantum electrodynamics. Renormalisation strategies were of no use, unless...
- Spin-1 bosons follow the non-commutative gauge field theory proposed by Yang and Mills in 1954, and...
- The gauge invariance is spontaneously broken by the Higgs mechanism.
By integrating all of these properties, scientists arrive at the Standard Model of electroweak interactions, which agrees with experiments and is now universally accepted.
Strong interactions
Since the 1950s, many new particles have been produced from high-energy collisions. The notion that "all matter is composed of protons, neutrons, and electrons" has been completely shattered. The subsequent developments can be divided into several stages.
- The first stage involved classifying particles, similar to the way in which Mendeleev summarised the chemical elements into the Periodic Table. Particles were classified according to flavour symmetries, such as
.
- The next step was to explain these properties. The theory of quarks was proposed by Gell-Mann and Zweig. Quarks carry a charge of
units, and have never been directly observed. Some (including Gell-Mann at the time) believed that quarks were just mathematical entities and not physical particles. Others believed (now known to be correct) that quarks are real and have "color". The color charge coupling constant increases with distance, so that at long distances (in the "infrared" limit), quarks are permanently confined and cannot appear independently. However, why does the coupling constant increase at long distances?
- From electron-proton scattering, scientists discovered that quarks are almost free at short distances (in the "ultraviolet" limit). They took this to mean that the coupling constant decreases at shorter distances, resulting in asymptotic freedom in the "ultraviolet" limit.
- The variation of the coupling constant with distance depends on the renormalisation group
function. If
, the coupling constant decreases at large distances (inconsistent with experiment); if
, the coupling constant increases at large distances (which explains infrared confinement and ultraviolet freedom). People have calculated the
function according to various theories and found (almost) without exception that
.
- Gross, Wilczek, and Politzer calculated the
function for Yang–Mills theory in the early 1970s and found it to be negative, thereby in one fell swoop resolved many outstanding problems, triggering a revolution in field theory. The strong interaction was subsequently incorporated into Yang–Mills theory (resulting in quantum chromodynamics, or QCD), and over the course of the following 50 years, scientists have refined the theory with increasingly precise predictions. The property
is found only in Yang–Mills theory, thereby uniquely explaining infrared confinement and ultraviolet asymptotic freedom. I have used the Chinese phrase "dazzling with the violet blossoms and coyly hiding the red petals" to describe the situation [2].
The discovery of the distinction between right- and left-handed spins (i.e. non-conservation of parity) and the Yang–Mills theory form the cornerstone of elementary particle theory in the past few decades. Today, Yang–Mills theory has become a core concept in modern physics. These two achievements are discussed separately below. (This part not in the present translation.)
3. Biography of Professor Yang Chen-Ning
3.1 Birth and early life
Yang Chen-Ning was born in Hefei, Anhui in the autumn of 1922. In the specific date of his birth lies an interesting tale. Yang Chen-Ning was born on the 11th day of the eighth month according to the Chinese lunar calendar. In those days, hardly anyone paid attention to the Gregorian calendar. However, when he was preparing to go abroad to study after World War II, he needed to apply for a passport, for which the birthday according to the Gregorian calendar was required. Based on an estimate, he just put down 22 September . Years later, it was found that the 11th day of the eighth lunar month in 1922 corresponds to 1 October – the same day as our National Day. Coincidentally, Professor Yang’s two most important papers were both published on 1 October in the journal Physical Review: Yang–Mills gauge theory on 1 October 1954, parity non-conservation on 1 October 1956. I discovered this coincidence 20 years ago and spoke about it on the occasion of Professor Yang’s 80th birthday [2].
Yang Chen-ning’s father, Yang Wu-Chih, graduated from Peking Normal College and worked as a mathematics teacher in Anhui. About a year after Chen-Ning was born, Yang Wu-Chih obtained a government scholarship for doctoral studies at the University of Chicago. In 1928, when Yang Chen-Ning was six years old, he returned to China. He first taught at Xiamen University, then in 1929 accepted an offer from Tsinghua University. Thus, at the age of seven, Yang Chen-Ning moved to Tsinghua, which naturally has a special place in his heart, and which is now his home.
Following the Japanese invasion and the fall of Beijing in 1937, fifteen-year-old Yang Chen-Ning retreated to Kunming with his parents, passing through Guangzhou, Hong Kong and Vietnam. His father taught at National Southwest Associated University, a war-time confederation among Peking University, Tsinghua University and Nankai University. National Southwest Associated University employed some of China’s leading academics (the teaching staff included the famous historian and philosopher Ch'ien Mu who would be known to students of CUHK). In just eight years from 1938 to 1946, the university cultivated a lot of talents, including the scientists eventually responsible for the development of China’s first atomic and hydrogen bombs as well as the satellite programme, and over a hundred academicians in the Chinese Academy of Sciences and the Chinese Academy of Engineering.
Yang Chen-Ning completed his undergraduate studies at National Southwest Associated University. Upon graduation in 1942, he stayed for a master's degree (officially registering as a postgraduate student of Tsinghua). In 1944, he was awarded a Boxer Indemnity Scholarship for study in the United States, though his departure was delayed for one year until the war ended.
3.2 Study abroad and early research work
Yang Chen-Ning’s choice of the University of Chicago was not motivated by a desire to follow in his father's footsteps; rather, he wanted to study under the famous Enrico Fermi. Fermi was a pioneer in nuclear fission research, and Chicago was also home to the world’s first nuclear reactor that successfully produced a chain reaction. Fermi was proficient in both theoretical and experimental physics, but Yang believed that he was not suited to experimental physics, and chose Edward Teller as his PhD supervisor. Teller was no less famous than Fermi – at the time, the most commonly used weak interaction theory was called the Fermi-Teller interaction. Teller ultimately devoted himself to hydrogen bomb research and was known as the "the father of the hydrogen bomb" in the United States.
As Professor Yang has mentioned on many occasions, those years were a great time to be a young scientist working on particle physics. The discovery of new particles led to a range of phenomena that could not be explained by prevailing theories, presenting physicists with exciting opportunities. (In the framework of Kuhn [1], this was an era of scientific revolution.) Some people might say that Yang was lucky to have chosen a branch of physics that was poised to take off. However, more likely the young Yang Chen-Ning had better insight into the emerging trends than many of his peers. It is this kind of insight and judgment that made him encourage young people to focus on novel fields ready for rapid advance.
After obtaining his PhD, Yang Chen-Ning continued his research at the Institute for Advanced Study (IAS) at Princeton , where Albert Einstein was also working. Yang’s years at IAS were the golden age of his scientific career. His most notable achievements were the non-conservation of parity (1956) and Yang–Mills gauge theory (1954). He also made important contributions in other areas such as statistical physics. He was best known for his 1956 work, because his proposal on parity violation attracted immediate attention and was quickly confirmed by experiments. Yang and Lee Tsung-Dao were awarded the Nobel Prize the following year in recognition of their work. In contrast, his 1954 work on gauge fields was so much ahead of the times that it did not receive widespread attention for more than a decade – it was not until the late 1960s and even 1970s when gauge theory became a core part of theoretical physics. These two theories will be discussed in more detail below. (This part not included in the present excerpt.) In 1966, Yang Chen-Ning moved to the State University of New York (SUNY) at Stony Brook, where he was appointed to the Albert Einstein Chair.
Einstein’s Nobel Prize-winning work was not his most famous and influential theory of relativity (special or general relativity), and in the same way that Yang Chen-Ning did not receive the Nobel Prize for his most famous and influential work on gauge theory.
3.3 Relationship with CUHK
Yang’s scientific contributions belong to all of humanity. Nevertheless, he retains a very strong sense national and cultural identity, clearly articulated in his Nobel Prize Banquet speech [3]. Today, we must also share the story of his long-standing relationship with CUHK.
As mentioned above, during the Sino-Japanese War, Yang Chen-Ning travelled to Kunming via Hong Kong and Vietnam. He has not spoken much about his memories of passing through Hong Kong. After all, he was only fifteen at the time, and with his parents retreating in haste from the battlefront of war.
The next time he set foot in Hong Kong was in 1964, 27 years later, to meet his parents. His parents were living in Shanghai at the time, and his father was a professor of mathematics at Fudan University. In the 1960s, relations between China and the United States were worse than they are today. As a result, Yang would have found it difficult to travel to Shanghai, and it would have been even more challenging for his parents to travel to the US. CUHK, established just a year earlier, was aware of his wishes and invited Professor Yang to visit and give a public lecture. Our long-standing association with Yang Chen-Ning dates from the university’s earliest days.
Of course, back then there was no MTR station or the Sha Tin campus as we know it today. The railway was not electrified, and there was only an hourly diesel train service. The train station was called Ma Liu Shui – it was not until several years later that it would be renamed as University Station. The current campus had yet to be built, and the only academic buildings on site were those belonging to Chung Chi College. The other two constituent colleges were scattered across Hong Kong Island and Kowloon, and the University’s offices were temporarily located in the Hang Seng Bank Building in Mong Kok. Yang’s public lecture was held in the newly opened Hong Kong City Hall.
I digress about the University’s challenging beginnings because young people need to appreciate the tremendous societal changes that have taken place in Hong Kong over the past decades. Of course, the example I give is just a minor anecdote. Nevertheless, in the following section I will talk about Yang's personal experiences and insights into China’s history over the past century, and it is important to be able to place those accounts into the proper historical context in order to understand his thinking.
I am in a good position to speak about the 1964 public lecture, as I had the good fortune to be among the audience. I was a high school student, just like many of you here today. In order to ensure a seat, I started queuing very early. However, after listening to the afternoon lecture, I would have to admit I understood very little. There was no Internet back then, so high school students’ knowledge of science was restricted to a few textbooks. Nevertheless, his lecture provided an important first glimpse into the world of scientific research and illustrated the profound, universal and exciting nature of the natural phenomena that scientists investigate. Recently, we came across a recording of his 1964 lecture, with excellent sound quality. The CUHK Department of Physics has supplemented the audio recording with modern illustrations, and the lecture has been made available online in video format [4].
Twelve years later, in 1976, Professor Yang returned to CUHK to give another lecture. By then, the CUHK campus had begun to take shape. The lecture was held in front of a packed audience at the Science Centre in lecture hall L1, and covered gauge fields, magnetic monopoles, and fibre bundles. I was again fortunate to be present. By that time, I was a young teacher in the Department of Physics, and my area of research was closely related to Professor Yang's topics, so I had no problems understanding the concepts he discussed. (Over the past few years, I have been teaching an electrodynamics course for post-graduates, and one week of the course is dedicated to these topics.)
During the late 1970s and 1980s, Professor Yang came to CUHK more frequently to give lectures. He has been an Honorary Professor since 1983 and a Distinguished Professor-at-Large since 1986. He has also given numerous physics lectures and public speeches, including "Physics and Symmetry" in 1982 and "Forty Years as a Student and a Teacher" in 1983. Professor Yang was awarded an honorary doctorate in 1998, and taught a postgraduate elective course in 1983. In 2006, he designed and taught a special elective course called "Thematic Melodies of 20th Century Theoretical Physics".
In 1998, Yang Chen-Ning made an important decision – he donated all the documents, letters, notes and manuscripts prepared and collected over the decades, plus the medals he had won (including his Nobel medal) – to CUHK. In the wake of this decision, the university established the CN Yang Archive, a miniature museum that documents Yang’s seminal contributions to science. The Archive is located in Tin Ka Ping Building, next to the library’s Rare Book Collection. The collection is open to visitors. (Due to capacity limitations, visitors are required to register in advance.)
From the 1980s and for over a decade, Professor Yang worked at CUHK for nearly three months each year , living in the university’s staff residence. His office was located in the Science Centre.
3.4 Return to Tsinghua
In 2003, at the age of 81, Professor Yang decided to leave Stony Brook in New York and to return to Tsinghua, the picturesque campus where he had spent his childhood. However, he did not retire – since his return, he has led the Institute for Advanced Study, where he has continued to make contributions to Chinese science by recruiting and mentoring highly qualified young scholars. His sentiments are reflected in the poem by Zhu Ziqing that he quoted during his retirement speech at the State University of New York [5]:
“The sunset is infinitely good, so why be melancholy about dusk.”
(Note: This sentence was inspired by a line from the Tang dynasty poet Li Shangyin: "The sunset is infinitely good, but it's almost dusk.")
In July 2017, the 9th Joint Meeting of Chinese Physicists Worldwide (OCPA9) was held in Beijing. The plenary session was held in the Grand Auditorium at Tsinghua University, and Professor Yang gave a half-hour keynote speech. During his speech, the 95-year-old Yang recalled that his childhood home was just a stone’s throw away, and that he used to sneak into the auditorium to play when he was seven. More than 80 years later, the auditorium is now air-conditioned and brightly lit, but much remains the same, exuding a quaint atmosphere and a sense of history. However, Chinese physics, science, and society in general have all undergone fundamental changes.
In 2007, Yang Chen-Ning wrote the following paragraph in the Foreword to his new book Light at Dawn [6]:
"The era of Lu Xun, Wang Guowei, and Chen Yinke was a long night in the history of the Chinese nation. I, together with my classmates from National Southwest Associated University, grew up during this endless long night. Fortunately, the Chinese nation has finally passed this long night and now stands at the dawn. I’m 85 years old and I won’t live to see the sun in its full glory..."
Ten years later, in 2017, Professor Yang and his wife Weng Fan co-authored another book with the self-evident title Morning Light [7]. The above words are repeated in the Foreword, followed by:
At that time, I felt... I saw the first glimmers of dawn, and I was afraid we’d have to wait another 30 years to see the sun in its full glory... I didn't expect that in the next 10 years, we’d see such enormous changes in China and in the rest of the world. Although the sun hasn’t fully risen, we can now see the first rays of sunlight.... It seems...I might live to see the sun after all!
4. Concluding remarks
Around a hundred years ago, the advent of quantum mechanics heralded a new era for physics, and great progress was made in particle physics. Weak interaction distinguishes left and right, and all interactions are described by gauge theory. Professor Yang’s work played a pivotal role in this story, with his gauge theory forming the basis for work on the unification of the fundamental interactions. For the physics community, the search for a Grand Unified Theory (GUT) can be likened to the aspiration of "seeing the sun in its full glory".
As Yang himself has observed, "China and the rest of the world have undergone enormous changes. …I might live to see the sun after all!"
Thanks to the great achievements made by physicists and the enormous changes that have taken place in China and the rest of the world over the past century, the prospects for the future are bright. As Professor Yang steps into his hundredth year, these hopes for the future are the best birthday gift for him.
Acknowledgements
My thanks to WANG Yi, SHEK Hiu Fung, CHU Ming-Chung, IU Kwan Sai, and FU Pengpeng for their valuable comments and help with preparing this text.
References
- Thomas S Kuhn, The Structure of Scientific Revolutions, 3rd ed. University of Chicago Press (1966).
- Kenneth Young, "Congratulations to Professor Yang Chen-Ning on his Eightieth Birthday", pp 266-267 in Ye Zhongmin The Story of Physicist Yang Chen-ning (Renqing Wuli Yang Chen-Ning), The Chinese University of Hong Kong Press (2002).
- CN Yang. "Speech at the Nobel Banquet", December 10, 1957. p. 247 in CN Yang, Selected Papers 1945 – 1980, with Commentary. Freeman & Co. (1980).
- https://cnyangarchive.cuhk.edu.hk/LectureVideo.aspx
- Yang Chen-Ning, "Response to Retirement Dinner" (1999), in [6], pages 294–297.
- Yang Chen-Ning and Weng Fan, Light at Dawn (Xu Guang), Joint Publishing Hong Kong (2008).
- Yang Chen-Ning and Weng Fan, Morning Light (Sun Xi), The Commercial Press (2018).
Authors:
Prof Kenneth YOUNG, Emeritus Professor, Department of Physics, The Chinese University of Hong Kong
November 2021